Methane Gas Hydrate Reservoirs
Methane hydrates are crystalline solids formed when water molecules enclathrate gas molecules. Methane hydrates are stable at high pressures and low temperatures. When warmed or depressurised, they decompose into gas and water. In nature, methane hydrates typically occur in permafrost regions and marine sediments. The motivation for research in methane hydrates is multifaceted. Methane hydrates are seen as an attractive future energy resource. It is estimated that the total carbon content of methane hydrates is possibly larger than the combined carbon content of all other fossil fuels. There are environmental concerns that the rising sea temperatures may destabilise marine gas hydrate with serious impacts on global climate and carbon cycle. There are concerns regarding the geotechnical risks of natural as well as human induced gas hydrate destabilisation, such as, rapid consolidation and seefloor subsidence, soil fluidisation, local and regional slope instability, etc.
From a modelling and simulation perspective, methane hydrates are one of the most complex porous geosystems. Methane hydrate reservoirs are typically characterized by large material heterogeneities, local anisotropies, and a large number of strongly coupled multiphysics processes, like multiphase multicomponent fluid flow, complex phase changes, fluid-solid interactions influenced by the evolving solid phase, thermal effects, and soil deformations, etc.
Mathematical model and numerical simulation
We have developed a thermo-chemo-hydro-geomechanical (TCHM) model which broadly considers the following phenomenological processes on a continuum scale: - non-isothermal and miscible water and methane flow (Darcy flow approximation) through porous soil matrix - equilibrium phase changes between methane and water (fluid ↔ fluid); vanishing phases, - non-equilibrium phase change of methane hydrate (solid ↔ fluid), - effect of hydrate phase change on the hydraulic properties of the porous matrix, - dissolved salt transport and related thermodynamic couplings with fluid transport and methane hydrate phase change, and - elasto-plastic poro-mechanical deformation of the soil matrix (within the small-displacement approximation).
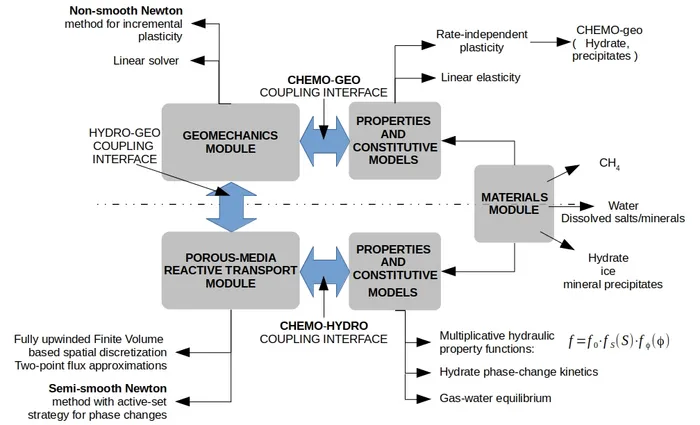
The model is decomposed into two blocks: 1) transport-block, and 2) geomechanics-block.
The transport-block models the non-isothermal reactive transport of methane, water, salt, and methane hydrates. A particular emphasis is laid on the consistent treatment of the equilibrium and non-equilibrium phase changes within the model. The non-equilibrium hydrate phase change is modeled as a first order kinetic process. The water-gas phase transitions like methane dissolution and water evaporation are formulated as a set of local inequality constraints which are directly integrated into the nonlinear solver using a nonlinear complementarity function.
The geomechanics-block models the elasto-plastic (or linear elastic) deformation of the sediment. The model is formulated based on the assumption that the hydrate and the soil form a single composite continuum phase at the macro-scale. The soil grains form the primary load-bearing skeleton, while the hydrate enhances the mechanical properties of this primary skeleton. We introduce a two-porosity concept: the total porosity, which is the void space enclosed by the primary soil skeleton, and the apparent porosity, which is the actual void volume available for fluid flow. The stress-strain behavior of the composite hydrate-soil matrix is modeled based on the Drucker-Prager plasticity theory for frictional granular materials. A small-strain rate-independent I1-J2 plasticity module is implemented within our numerical framework, which is enhanced with templates for general strain hardening material laws. The coupling between the two model blocks is introduced through an iterative block Gauss-Seidel scheme. We have also developed multi-rate time-stepping schemes to exploit the difference in time
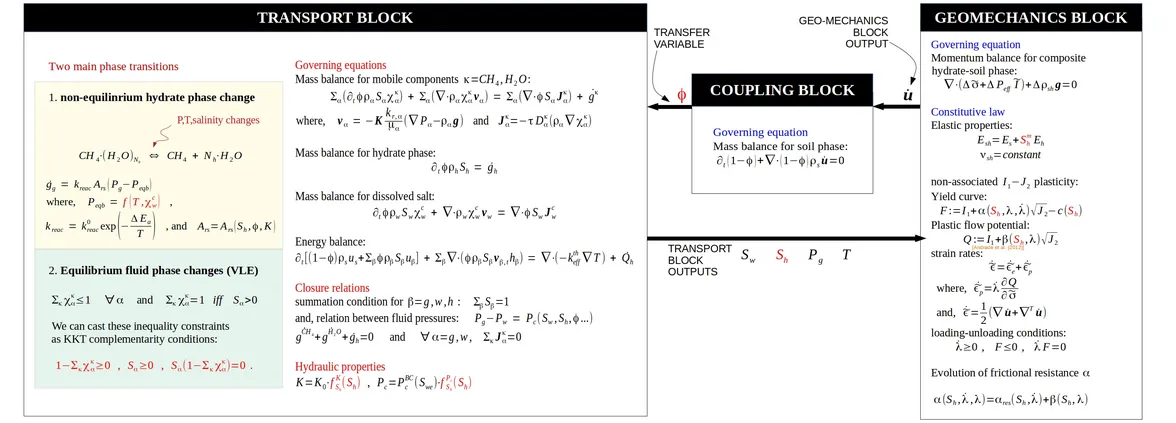
Selected results
One of the major accomplishments of this work has been the testing and validation of our TCHM model through highly controlled but detailed laboratory experiments conducted by the group of Dr. Matthias Haeckel at GEOMAR (Helmholtz Center for Ocean Research, Kiel) within the SUGAR Gas Hydrate project. We have also developed, parameterized and analyzed the constitutive material models for the geomechanical behavior of homogeneous gas hydrates based on the triaxial experiments, also conducted by the group of Dr. Matthias Haeckel. We are currently applying our methane hydrate model to study the problem of gas migration through the gas hydrate zones driven by sedimentation over paleo time scales in the highly dynamic geological setting of the Black Sea.